Developing porous copper/aluminium-chitosan biosorbent hydrogel beads for the removal of phosphate from wastewater
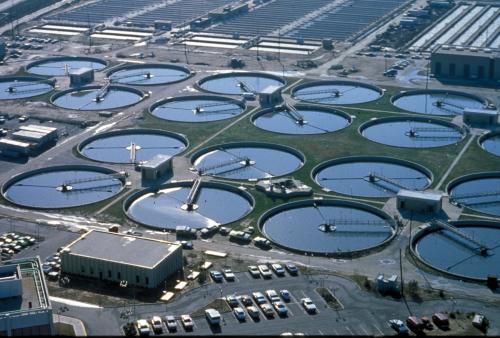
Downloads
Excess phosphorus is one of the principal causes of eutrophication, which causes severe ecological imbalance and harm to human health. In this study, several chitosan (CS)/copper and aluminum (CNT, ACH) hydrogel beads were created and tested for phosphorus removal. Further Microcrystalline cellulose and Cellulose Nano Fiber were also used to create stable CS/CNT - ACH hydrogel beads. The optimized CNT/CS settings with 0.2 mg CNT demonstrated outstanding removal efficacy. It effectively removed phosphate from an aqueous solution with a pH range of 4.5-5.5 using a completely mixed batch of 0.01M sodium dihydrogen phosphate, with 80% phosphate absorption achieved after 48 hours of contact time. The measured maximum adsorption capacity at pH 5.5 solution was 11.39 mg per 0.0206g of the beads (10 beads). The FTIR study revealed that all three varieties of synthesized beads have a healthy microstructure. Furthermore, the findings of the kinetic study indicated a low absorption rate at 15ºC and a moderate absorption rate at 45ºC. The adsorbent efficiently removed phosphate during 12 hours of contact time, according to a batch adsorption study, using 20 beads weighing 0.0412g. Electrostatic attraction and ion exchange can both be responsible for phosphate absorption. Furthermore, 10 of the 21 control MCC and CNF beads could remove more than 60% phosphate after 48 hours of contact time with identical solute distributions. This adsorbent might be deployed to effectively treat phosphorus-contaminated water to prevent eutrophication.
Banc C, Gautier M, Blanc D, Lupsea-Toader M, Marsac R, Gourdon R. Influence of pH on the release of colloidal and dissolved organic matter from vertical flow constructed wetland surface sludge deposits. Chemical Engineering Journal. 2021 Aug 15;418:129353.
International Environmental Technology 2022, ‘How Do Sulphates Get into Water?’, Envirotech Online, Environmental, viewed 17 June 2022, <https://www.envirotech-online.com/news/water-wastewater/9/breaking-news/how-do-sulphates-get-into-water/48645>.
Deyab, M., El-Adl, M., Ward, F., & Omar, E. (2021). Trophic status, phytoplankton diversity, and water quality at Kafr El-Shinawy drinking-water treatment plant, Damietta. AQUA—Water Infrastructure, Ecosystems and Society, 70(3), 342-360.
Almanassra, I. W., Mckay, G., Kochkodan, V., Atieh, M. A., & Al-Ansari, T. (2021). A state of the art review on phosphate removal from water by biochars. Chemical Engineering Journal, 409, 128211.
Ruzhitskaya, O. A., & Ponomareva, N. S. (2019). Modern biological and biological-chemical methods for removing phosphates from wastewater. Systems Technologies, (3 (32)), 18-22.
Silva A., Richard C., Bessodes M., Scherman D., Merten O. Growth Factor Delivery Approaches in Hydrogels. Biomacromolecules. 2009;10:9–18. doi: 10.1021/bm801103c. [Google Scholar]
Ruso, J. M., & Messina, P. V. (2017). Application of natural, semi-synthetic, and synthetic biopolymers used in drug delivery systems design. In Biopolymers for medical applications (pp. 46-73). CRC Press.
Nele V., Wojciechowski J.P., Armstrong J.P.K., Stevens M.M. Tailoring Gelation Mechanisms for Advanced Hydrogel Applications. Adv. Funct. Mater. 2020;30:2002759. doi: 10.1002/adfm.202002759.
Raghavan S.R., Fernandes N.J., Cipriano B.H. Shape-Changing Tubular Hydrogels. Gels. 2018;4:18. doi: 10.3390/gels4010018.
Macaya D., Spector M. Injectable hydrogel materials for spinal cord regeneration: A review. Biomed. Mater. 2012;7:012001. doi: 10.1088/1748-6041/7/1/012001
Van Tran, V., Park, D., & Lee, Y. C. (2018). Hydrogel applications for adsorption of contaminants in water and wastewater treatment. Environmental Science and Pollution Research, 25, 24569-24599.
Yang, Y., Wu, N., Li, B., Liu, W., Pan, F., Zeng, Z., & Liu, J. (2022). Biomimetic porous MXene sediment-based hydrogel for high-performance and multifunctional electromagnetic interference shielding. ACS nano, 16(9), 15042-15052.
Hu X., Ricci S., Naranjo S., Hill Z., Gawason P. Protein and Polysaccharide-Based Electroactive and Conductive Materials for Biomedical Applications. Molecules. 2021;26:4499. doi: 10.3390/molecules26154499.
Mahmood, A., Patel, D., Hickson, B., DesRochers, J., & Hu, X. (2022). Recent progress in biopolymer-based hydrogel materials for biomedical applications. International Journal of Molecular Sciences, 23(3), 1415.
Xi, H., Li, Q., Yang, Y., Zhang, J., Guo, F., Wang, X., ... & Ruan, S. (2021). Highly effective removal of phosphate from complex water environment with porous Zr-bentonite alginate hydrogel beads: Facile synthesis and adsorption behavior study. Applied Clay Science, 201, 105919.
Moharrami, P., Hazrati, S., Shakeri, F., Motamedi, E., & Ariaeenejad, S. (2024). Sodium alginate-and chitosan-based hydrogels with different network charges for selective removal of cationic and anionic dyes from water. Water Quality Research Journal, 59(4), 205-222.
Kluczka, J., Dudek, G., Pudło, W., Kazek-Kesik, A., & Turczyn, R. (2021). Boron Removal by Sorption on Modified Chitosan Hydrogel Beads. Materials 2021, 14, 5646.
Gupta, P. K., Raghunath, S. S., Prasanna, D. V., Venkat, P., Shree, V., Chithananthan, C., ... & Geetha, K. (2019). An Update on Overview of Cellulose, Its Structure and. Cellulose, 59.
Awang Wahab, D. N., M. B. M. Siddique, N. Khairuddin, J. J. Chew, and H. T. Su. (2024). Mechanical, structural and barrier properties of starch-based film reinforced with cellulose microfibres extracted from midribs of Musa Saba'. Food Research, 8 (3),117-123.